The first step toward a cure is a treatment, and the first step toward a treatment is a research roadmap.
Executive Summary
The goal of the FBXO31 Cure Roadmap is to accelerate the identification of a treatment modality for patients with FBXO31 D344N mutation whose parent-led foundation commissioned the development of this Roadmap. This work is expected to enhance our understanding of FBXO31 function and its impairments leading to new treatment options for FBXO31-related neurological disorders and potentially even for neurodevelopmental disorders associated with cerebral palsy.
Our key recommendations for the next 12-24 months are:
- Initiate a drug repurposing screen on patient iPSC-derived neural progenitor cells to identify clinically actionable repurposable hits. This effort driven by Perlara is expected to yield repurposable hits that can be translated into so-called “1-to-N” trials (coordinated, parallel single-patient studies expanded to broader patient communities).
- Build a drug repurposing consortium that would bring together a broader coalition of clinicians and researchers supported by the FBXO31 Foundation. The goal of this consortium is twofold: (a) enable the sharing of patient information and biosamples, and (b) delineate the role of repurposed drugs in alleviating FBXO31 pathology at a cellular level. It’s expected that these studies would be invaluable for accelerated drug development.
- Additionally explore alternative drug development opportunities for FBXO31 D344N variant, including ASO (antisense oligonucleotides), PROTAC (proteolysis-targeting chimeras), and other gene therapy approaches.
- Increase awareness of FBXO31-related neurological disorder, particularly via centers for neurological diseases or specializing in cerebral palsy. It’s expected that awareness leading to more sequencing efforts could further enhance the patient pool with FBXO31 variants.
Introduction
Disease Overview: Basic science
FBXO31 and Cell cycle: The cell cycle is a precisely timed series of events fundamental to normal cellular function and organismal health1. Progression through the different phases of the cell cycle is tightly regulated by specific checkpoints and controlled by regulatory proteins including cyclins and cyclin-dependent-kinases (CDKs)2. Aberrations in cell cycle mechanisms lead to an array of diseases, from cancers to neurodegenerative disorders.
The ubiquitin-proteasome system (UPS) plays a critical role in regulating the cell cycle by targeting specific proteins for degradation. The UPS consists of three subunits: E1 (ubiquitin-activating), E2 (ubiquitin-conjugating) and E3 (ubiquitin ligase), that activate ubiquitin and subsequently transfer it to the protein destined for degradation3. Specifically, UPS regulates transitions between the cell cycle phases via cyclin degradation, consequently tightly regulating the levels of cyclins and associated cyclin dependent kinases2. This system ensures that cellular function and genomic integrity are maintained3.
FBXO31 (F-box only protein 31) was discovered as a tumor suppressor factor in 20054. The F-box proteins (FBPs) are a diverse group of proteins characterized by the presence of a conserved F-box motif of ~40 amino acids5. This motif allows the protein to interact with SKP1 (S-phase kinase-associated protein 1) and constitutes the SCF (SKP1-Cullin-F-box protein) E3 ubiquitin ligase complex. The SCF complex is instrumental in targeting proteins for ubiquitin-mediated degradation, thereby regulating various cellular processes such as cellular proliferation, cell cycle progression, and apoptosis. To date, there are over 600 E3 ligases that have been identified, of which the SCF or Cullin-RING E3 ligase (CRL) family is the largest, with CRL1 being the most characterized5–7.
FBXO31, belonging to the FBXO subfamily of F proteins, is expressed across tissue types with particularly high expression in the brain and is highly expressed in the G2→G1 phase of the cell cycle4. It has a well-defined role in DNA damage response and tumorigenesis. Acting as a tumor suppressor (alterations causing loss-of-function effects)8, FBXO31 has been implicated in breast cancer4,9, melanoma10,11, hepatocellular carcinoma12, gastric cancer13,14, and glioma15. Conversely, it functions as an oncogene (alterations causing gain-of-function effects) in esophageal squamous cell carcinoma16 and lung cancer17.
FBXO31’s role in the DNA damage response includes halting cell cycle progression to allow for damage assessment and repair, particularly through pathways involving Ataxia telangiectasia mutated kinase (ATM)18, DNA-dependent protein kinase (DNA-PK), and Ataxia telangiectasia and Rad3-related protein (ATR) that maintain genomic stability by detecting DNA double-strand breaks by homologous recombination and non-homologous end joining, and in response to replication stress and single-strand breaks respectively19. In response to genotoxic stress, FBXO31 facilitates cyclin D1 degradation20.
Substrate specificity and neuromodulation of FBXO31: Cyclin D1, a substrate of SCFFBXO31, plays a pivotal role in initiating and advancing the cell cycle through the G1 phase in response to external stimuli20,21. Considered a proto-oncogene22, changes in the ubiquitination process and the subsequent stability of cyclin D1 have been identified as cancer biomarkers 23, indicative of the progression of the disease.
An unconventional function for cyclin D1 in neuronal stem cells is its effect on cell fate influencing whether cells proliferate or differentiate by regulating the G1 phase24. In pathological contexts, nuclear cyclin D1 can prompt apoptosis in post-mitotic neurons25. Interestingly, cyclin D1’s presence in the cytoplasm of differentiating neurons and neuroblastoma cells suggests roles in preventing apoptosis or promoting cell cycle withdrawal26. Additionally, cytoplasmic cyclin D1 in the cytoplasm supports neuritogenesis in response to nerve growth factor (NGF) and contributes to neuronal plasticity during hippocampal development27,28. More recently, cyclin D1 was identified to modulate the activity of Gamma-aminobutyric acid (GABA), a crucial neurotransmitter in the brain, and thereby neuronal inhibition29. Deficits in GABA receptor function are implicated in various neurological disorders, from anxiety to epilepsy, with a significant role in synaptic inhibition and neurodevelopment in the hippocampus and cortex30,31.
An emerging role for FBXO31 points towards it being a regulator of neuronal morphogenesis and polarity32. These two distinct processes create the information flow necessary for brain functions such as memory, learning, and emotion. In addition to Parkinson’s disease protein Parkin I, FBXO31 is one of few E3 ubiquitin ligases that localizes to the centrosome, the microtubule organizing center, that regulates neuronal polarity, spindle formation motility and adhesion crucial to cell division32.Malfunctioning centrosomes potentiate cortical defects during development (the region of the brain associated with sensory, and motor development)33,34. The ligase activity of FBXO31 drives neuronal morphogenesis and is attributed to axon and dendritic growth, and axon specification. FBXO31 also targets centrosome polarity protein Par6c for degradation35. Reduction in Par6 degradation by another E3 ligase (Smurf1) drives the formation of a Par3/Par6/aPKC complex36,37 and thereby axon specification and polarity. Conversely, FBXO31 drives the proteasomal degradation of Par6c (the most abundant Par6 protein) regulating axonal growth and neuronal polarity32.
These examples underscore the significance of FBXO31 in maintaining cellular function, supporting normal development, and potentially influencing the pathogenesis of neurodegenerative diseases. Other key FBXO31 substrates include MCM7, MDM2, CDT1, FOXM1, Slug, Histone H2AX, TRF1, FAT10 perturbations of which can impact neurogenesis, neuronal survival, synaptic plasticity, and in general brain development38.
It has been proposed that the conserved binding pocket of FBXO31 enables the selective recognition of C-terminal peptides with an amide group (CTAPs), thereby serving as a distinct marker for proteins targeted for degradation by FBXO31, including hormones and neuropeptides39. Mutations affecting the binding domain of FBXO31 disrupt this specificity, leading to the degradation of newly identified proteins lacking amidation, while proteins originally marked with CTAPs accumulate within the cell. This toxic gain-of-function mutation in FBXO31 affects the function of downstream substrates but also results in the accumulation of protein degradation products, thereby disrupting the cell cycle. Dysregulation of this process in tissues where FBXO31 is highly expressed such as the brain 4 and skeletal muscles40, can significantly impact the development of neuro and skeleton-muscular disorders.
Disease Overview: Clinical Features of FBXO31-related cerebral palsy
To date, there have been six reported cases of a neurodegenerative disorder related to cerebral palsy caused by mutations in FBXO3141. All affected patients have a de novo D334N (p. Asp334Asn) mutation. This mutation is located in the cyclin D1 binding region of FBXO31 and alters the electrostatic charge in the binding pocket, leading to reduced levels of cyclin D1 in these patients compared to controls42. Conflicting studies suggest that FBXO31 amelioration increases levels of cyclin D139. Modulation via CTAP and switching in electrostatic charge in the binding domain likely together alter cyclin D1 binding and induce substrate-related toxicity.
The characteristic effect of mutation is a form of brain injury categorized as cerebral palsy typified by poor coordination, stiff muscles, weak muscles, and tremors. Infants, heterozygous for mutation, are born full-term and are not diagnosed with abnormalities prenatally. Hypotonia, low muscle tone, is identified in neonates followed by a diagnosis of cerebral palsy. MRI scans reveal a reduction in white matter distribution and content in certain patients. Gross motor milestones such as rolling over, sitting, crawling or walking are delayed in infants. Speech impairments (primarily receptive language) and cognitive deficits including attention deficit hyperactivity disorder are present. Central and peripheral motor pathways are affected leading to spasticity and dystonia. Children present with global developmental delay and varying levels of intellectual disability. However, underlying motor or neurodevelopmental issues do not worsen over time. Literature reports one patient with intestinal malrotation in whom bowel resection was required (see Figure 1 on clinical presentation below)43 (includes observations by FBXO31 Foundation).
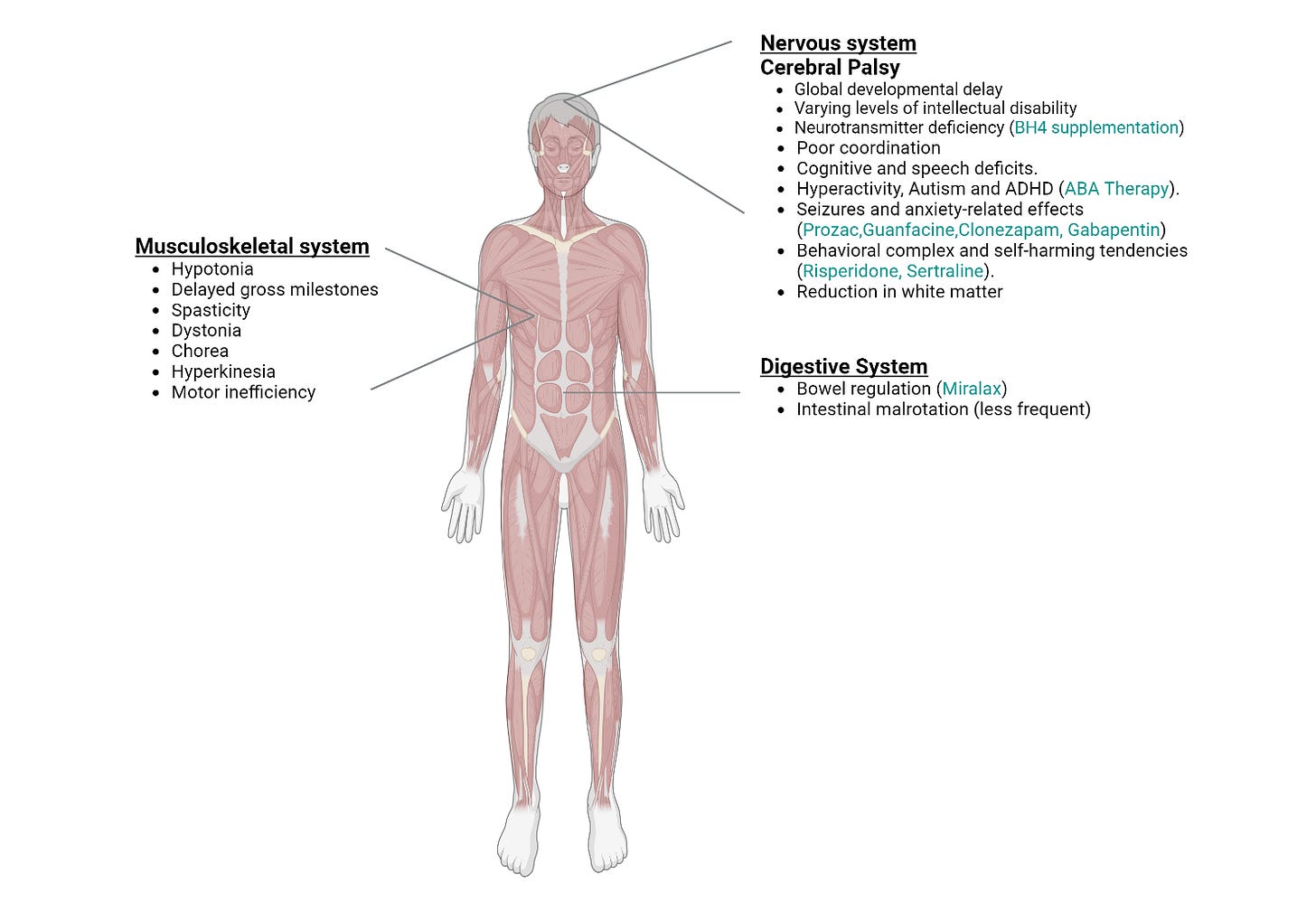
Recessive mutations in FBXO31 offer insights into a potential model for understanding the cerebral palsy phenotype associated with the D334N mutation. A 5-base pair deletion in FBXO31 results in a frameshift mutation that introduces a premature stop codon p.(Cys283asnfs*81). This mutation is characterized by dysmorphia and intellectual disability but does not manifest as cerebral palsy44. The truncation of the protein leads to its degradation via nonsense-mediated decay resulting in reduced levels of FBXO31. Consequently, this reduction prevents any potential toxic gain-of-function effects. Although decreased FBXO31 levels likely disrupt normal neuronal development, compensatory mechanisms mitigate the extent of damage44,45. This model posits that any intervention that corrects the gain-of-function toxicity will result in a significant correction of the pathophysiology. As such, there are many therapeutic approaches with the potential to positively impact the progression of FBXO31-related cerebral palsy (see Therapeutic Readiness).
Disease Models: Cellular Models
A crucial step in developing cures is establishing disease models to investigate disease mechanisms and test potential therapies. Reliable disease models provide essential evidence of safety and efficacy before clinical trials. The development of models here is essential for understanding how aberrant FBXO31 activity or lack thereof influences proteostasis and neurological dysfunction. Cellular models, the first to be developed, are relatively simple to generate and handle in vitro. These models are vital for large-scale therapeutic screenings and for exploring the activity and mechanisms of new treatments.
Developing a model system for FBXO31 is complicated by several factors:
- Substrate Interaction: FBXO31, like other F-box proteins, has multiple binding partners, making it difficult to delineate their interactions and downstream effects. Functional redundancy among F-box proteins46 can also obscure the specific effects of FBXO31 mutations.
- Cell-Type Specificity: FBXO31’s function varies across different cell types and tissues, making it challenging to develop models that accurately reflect its role in specific contexts, such as neuronal cells versus other cell types; developmental stages (during neuronal development vs post development).
- Model Organism Limitations: Common model organisms may not fully replicate human FBXO31-related disease phenotypes due to differences in gene expression, protein function, and developmental processes, limiting the model’s relevance.
This often requires the establishment of different types of cellular models to answer questions iterating FBOX31 dysfunction and therapeutics. Thanks to efforts in academic discovery science, however, several options exist to overcome these challenges.
Patient iPSCs are currently in development and will serve as the foundational research asset in drug repurposing screens, and beyond.
Disease Models: Whole-Organism Models
At present, there is a lack of published whole-organism models for FBXO31. Owing to their genetic similarity to humans, mouse models are indispensable for studying the pathological mechanism of human diseases, as well as for identifying new treatments. The FBXO31 mouse and Zebrafish model are currently under development and/or validation.
Prepared for Laura Avery and the FBXO31 Foundation
Authors:
- Mathuravani Thevandavakkam, PhD, Cure Guide and Director of Drug Repurposing, Perlara PBC
- Uche Medoh, PhD. Cure Guide, Perlara PBC & Principal Investigator at the Arc Institute
- Ethan O. Perlstein, PhD. CEO of Perlara PBC & Maggie’s Pearl, LLC & Curetopia